Improving Security in the Cloud; Hide and Seek Signals; A Supernova with a View
Improving Security in the Cloud
Less and less of today’s computing is done on desktop computers; cloud computing, in which operations are carried out on a network of shared, remote servers, is expected to rise as the demand for computing power increases. This raises some crucial questions about security: Can we, for instance, perform computations on data stored in “the cloud” without letting anyone else see our information? Research carried out at the Weizmann Institute of Science and the Massachusetts Institute of Technology (MIT) is moving us closer to the ability to work on data while it is still encrypted, giving an encrypted result that can later be securely deciphered.
Attempting computation on sensitive data stored on shared servers leaves that data exposed in ways that traditional encryption techniques can’t protect against. The main problem is that to manipulate the data, it has to be first decoded. “Until a few years ago, no one knew if the encryption needed for this sort of online security was even possible,” says Dr. Zvika Brakerski, who recently completed his PhD in the group of Prof. Shafrira (Shafi) Goldwasser of the Department of Computer Science and Applied Mathematics. In 2009, however, Craig Gentry, a PhD student at Stanford University, provided the first demonstration of so-called fully homomorphic encryption (FHE). But the original method was extraordinarily time-consuming and unwieldy, making it highly impractical. Gentry constructed his FHE system by using fairly sophisticated math, based on so-called ideal lattices, and this required him to make new and unfamiliar complexity assumptions to prove security. Gentry’s use of ideal lattices seemed inherent to fully homomorphic encryption; researchers assumed that they were necessary for the server to perform such basic operations as addition and multiplication on encrypted data.
Dr. Brakerski, together with Dr. Vinod Vaikuntanathan (a student of Prof. Goldwasser’s at MIT), surprised the computer security world earlier this year with two recent papers in which they described several new ways of making fully homomorphic encryption more efficient. For one thing, they managed to make FHE work with much simpler arithmetic, which speeds up processing time. And a surprise discovery showed that a mathematical construct used to generate the encryption keys could be simplified without compromising security. Gentry’s original ideal lattices are theoretical collections of points that can be added together—as in an ordinary lattice structure—but also multiplied. But the new research shows that the lattice does not have to be ideal, which simplifies the construction immensely. “The fact that it worked was something like magic, and it has challenged our assumptions about the function of the ideal lattices in homomorphic encryption,” says Dr. Brakerski.
Their result promises to pave a path to applying FHE in practice. Optimized versions of the new system could be hundreds—or even thousands—of times faster than Gentry’s original construction. Indeed, Drs. Brakerski and Vaikuntanathan have managed to advance the theory behind fully homomorphic encryption to the point that computer engineers can begin to work on applications. These might include, for instance, securing medical information for research: A third party could perform large medical studies on encrypted medical records without having access to the individuals’ information.
Prof. Shafrira Goldwasser’s research is supported by Walmart.
Hide and Seek Signals
The white blood cells that fight disease and help our bodies heal are directed to sites of infection or injury by “exit signs”—chemical signals that tell them where to pass through the blood vessel walls and into the underlying tissue. New research at the Weizmann Institute, which appeared in Nature Immunology online, shows how the cells lining blood vessel walls may act as “selectors” by hiding the signals where only certain “educated” white blood cells will find them.
In previous studies, Prof. Ronen Alon and his team in the Department of Immunology had found that near sites of inflammation, white blood cells rapidly crawl along the inner lining of the blood vessels with tens of tiny legs that grip the surface tightly, feeling for the exit sign. Such signs consist of migration-promoting molecules called chemokines, which the cells lining the blood vessels—endothelial cells—display on their outer surfaces like flashing lights.
In the new study, Prof. Alon and his team, including Drs. Ziv Shulman and Shmuel Cohen, found that not all chemokine signals produced by endothelial cells are on display. They observed the recruitment of subsets of immune cells called effector cells that are the “special forces” of the immune system: They receive training in the lymph nodes, where they learn to identify a particular newly invading pathogen and then return to the bloodstream on a search and destroy mission. Like the other white blood cells, effector cells crawled on tiny appendages along the lining of inflamed blood vessels near the site of pathogen entry, but rather than sensing surface chemokines, they used their legs to reach into the endothelial cells in search of the migration-promoting chemokines.
As opposed to the external exit signs, these chemokines were held in tiny containers—vesicles—inside the inflamed endothelial cell walls. The effector cells paused in the joins where several cells met, inserting their legs through the walls of several endothelial cells at once to trap chemokines as they were released from vesicles at the endothelial cell membrane. Once they obtained the right chemokine directives, the immune cells were quickly ushered out through the blood vessel walls toward their final destination.
The researchers think that keeping the chemokines inside the endothelial cells ensures, on the one hand, that these vital signals will be safe from getting washed away in the blood or eaten by various enzymes. On the other hand, it guarantees that only those effector cells with special training—those that can make the extra effort to find the signals—will pass through.
Says Prof. Alon: “We are now seeing that the blood vessel endothelium is much more than just a passive, sticky barrier—it actively selects which recruited cells actually cross the barrier and which will not. The endothelial cells seem to play an active role in showing the immune cells the right way out, though we’re not sure exactly how. Moreover, we think that tumors near blood vessels might exploit these trafficking rules for their benefit by putting the endothelial cells in a quiescent state or making the endothelium produce the “wrong” chemokines. Thus, immune cells capable of destroying these tumors will not be able to exit the blood and navigate to the tumor site, while other immune cells that aid in cancer growth will.”
Prof. Ronen Alon’s research is supported by the Kirk Center for Childhood Cancer and Immunological Disorders. Prof. Alon is the incumbent of the Linda Jacobs Professorial Chair in Immune and Stem Cell Research.
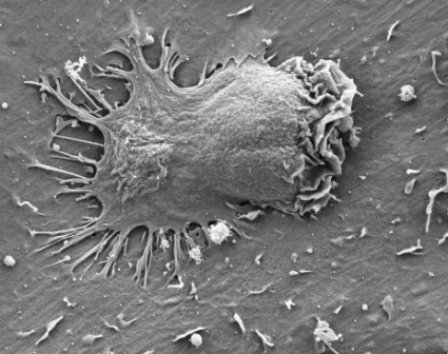
Electron microscope image of an effector cell inserting several appendages through endothelial cell membranes
Effector cells in an experimental control move on endothelium that does not produce internal chemokines
Effector cells, tagged green, seem to fade as they detect chemokines and move inward, past the surface of the blood vessel endothelium
A Supernova with a View
The closest supernova in 25 years yields new insights into its formation
Type 1a supernovae are such regular features of the Universe that astrophysicists use them to measure cosmic distances; however, we still don’t know exactly what makes these giant explosions occur. Now, scientists at the Weizmann Institute of Science, as part of an international effort to study supernovae, are beginning to clear up the mystery of why certain stars explode in a brilliant display at the ends of their lives.
New research began last August, when the automatic telescopes at the Palomar Transient Factory (PTF) in California that search for signs of developing supernova spotted one just a half a day into the explosion process. Not only was this a very early observation, but the supernova was in the Pinwheel Galaxy a mere 6.4 Megaparsecs away—the closest one in the last 25 years.
The scientists participating in PTF, including Drs. Eran Ofek and Avishay Gal-Yam of the Department of Particle Physics and Astrophysics, have recently published three new papers based on their initial observations and analysis, two of them appearing in Nature and one in The Astrophysical Journal.
Data on the new supernova came from x-ray and radio-wave telescopes, both Earth- and satellite-based. In addition, the researchers went over images of the Pinwheel Galaxy taken by the Hubble Space Telescope over the years to see if they could detect pre-explosion signs of the system that gave rise to the supernova.
To the scientist’s surprise, the x-ray and radio observations yielded no significant data, and the archival study did not reveal what was there beforehand. But, like the dog in the Sherlock Holmes story that didn’t bark, this lack turned out to be a significant clue: It allowed them to eliminate some of the various scenarios proposed for the type of setup causing the explosion.
These scenarios fall into two broad categories, both of them involving ancient, dense stars called white dwarfs. In one, two white dwarfs merge, and their combined mass becomes unstable, ending in a thermonuclear blast. In the other, the heavy white dwarf siphons off material from a companion star until it exceeds its stable weight limit, again causing an explosion. Proposed companion stars run the gamut from huge, gaseous red giants to smaller, Sun-like stars.
The team’s results, including an analysis of the material thrown off in the blast and of the “shock breakout” that takes place as the light released in the shockwave passes through the mass of erupting material (conducted by Itay Rabinak, a student of Prof. Eli Waxman of the same department), showed that the exploding star was, as predicted, a white dwarf: an extremely compact star with a diameter much smaller than that of our sun. And while the team didn’t manage to discount either category, they set an upper limit on the size of a possible companion, showing it could not have been a particularly large star, such as a red giant.
“Although we can’t rule out a white dwarf merger,” says Dr. Ofek, “our results point to another likely scenario in which a medium range star—close to our Sun’s size—supplied the white dwarf with the extra material needed to turn it into a supernova.”
Dr. Avishay Gal-Yam’s research is supported by the Peter and Patricia Gruber Award; the Nella and Leon Benoziyo Center for Astrophysics; the Yeda-Sela Center for Basic Research; and the Lord Sieff of Brimpton Memorial Fund.
Dr. Eran Ofek’s research is supported by the Willner Family Leadership Institute.