Fools’ Gold Found to Regulate Oxygen; Judging DNA by Its Cover; Locally Produced Proteins
Fools’ Gold Found to Regulate Oxygen
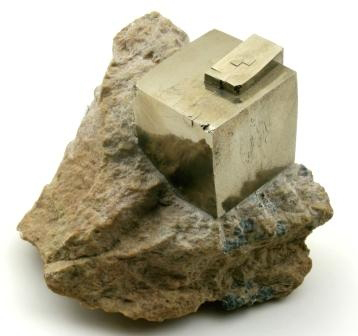
As sulfur cycles through Earth’s atmosphere, oceans and land, it undergoes chemical changes that are often coupled to changes in other such elements as carbon and oxygen. Although this affects the concentration of free oxygen, sulfur has traditionally been portrayed as a secondary factor in regulating atmospheric oxygen, with most of the heavy lifting done by carbon. However, new findings that appeared this week in Science suggest that sulfur’s role may have been underestimated.
Drs. Itay Halevy of the Weizmann Institute’s Environmental Science and Energy Research Department (Faculty of Chemistry), Shanan Peters of the University of Wisconsin and Woodward Fischer of the California Institute of Technology, were interested in better understanding the global sulfur cycle over the last 550 million years – roughly the period in which oxygen has been at its present atmospheric level of around 20%. They used a database developed and maintained by Peters at the University of Wisconsin, called Macrostrat, which contains detailed information on thousands of rock units in North America and beyond.
The researchers used the database to trace one of the ways in which sulfur exits ocean water into the underlying sediments – the formation of so-called sulfate evaporite minerals. These sulfur-bearing minerals, such as gypsum, settle to the bottom of shallow seas as seawater evaporates. The team found that the formation and burial of sulfate evaporites were highly variable over the last 550 million years, due to changes in shallow sea area, the latitude of ancient continents and sea level. More surprising to Halevy and colleagues was the discovery that only a relatively small fraction of the sulfur cycling through the oceans has exited seawater in this way. Their research showed that the formation and burial of a second sulfur-bearing mineral – pyrite – has apparently been much more important.
Pyrite is an iron-sulfur mineral (also known as fools’ gold), which forms when microbes in seafloor sediments use the sulfur dissolved in seawater to digest organic matter. The microbes take up sulfur in the form of sulfate (bound to four oxygen atoms) and release it as sulfide (with no oxygen). Oxygen is released during this process, thus making it a source of oxygen in the air. But because this part of the sulfur cycle was thought be minor in comparison to sulfate evaporite burial (which does not release oxygen) its effect on oxygen levels was also thought to be unimportant.
In testing various theoretical models of the sulfur cycle against the Macrostrat data, the team realized that the production and burial of pyrite has been much more significant than previously thought, accounting for more than 80% of all sulfur removed from the ocean (rather than the 30-40% in prior estimates). As opposed to the variability they saw for sulfate evaporite burial, pyrite burial has been relatively stable throughout the period. The analysis also revealed that most of the sulfur entering the ocean washed in from the weathering of pyrite exposed on land. In other words, there is a balance between pyrite formation and burial, which releases oxygen, and the weathering of pyrite on land, which consumes it. The implication of these findings is that the sulfur cycle regulates the atmospheric concentration of oxygen more strongly than previously appreciated.
Dr. Itay Halevy’s research is supported by the Sir Charles Clore Research Prize and the estate of Olga Klein Astrachan.
Judging DNA by Its Cover
Stem cells hold great promise for the medicine of the future, but they can also be a cause of disease. When these self-renewing, unspecialized cells fail to differentiate into diverse cell types, they can start dividing uncontrollably, leading to cancer. Already several decades ago, Weizmann Institute scientists were among the first to demonstrate the link between cancer and the faulty differentiation of stem cells. Now a new Weizmann Institute-led study, published in Molecular Cell, reveals a potential molecular mechanism behind this link.
The scientists managed to uncover the details of a step in the process of DNA “repackaging” that takes place during embryonic stem cell differentiation. It turns out that for the cells to differentiate properly, certain pieces of the packaging of their DNA must be labeled by a molecular tag called ubiquitin. Such tagging is required for turning on a group of particularly long genes, which enable the stem cell to differentiate. The researchers identified two switches: An enzyme called RNF20 enhances the tagging, whereas a second enzyme, USP44, does the opposite, shutting it down. Furthermore, it appears that both these switches must operate properly for the differentiation process to proceed efficiently. When the scientists interfered with the tagging – either by disabling the “ON” switch RNF20, or by deregulating the activity of the “OFF” switch USP44 – the stem cells failed to differentiate.
These experiments might explain the significance of molecular defects identified in a number of cancers, for example, the abnormally low levels of RNF20 in certain breast and prostate cancers and the excess of USP44 in certain leukemias. Notably, faulty differentiation of stem cells is often a hallmark of the more aggressive forms of cancer. This research was led by Prof. Moshe Oren of the Molecular Cell Biology Department, with Prof. Eytan Domany of the Physics of Complex Systems Department and Dr. Jacob Hanna of the Molecular Genetics Department. The team included Weizmann Institute’s Gilad Fuchs, Efrat Shema, Rita Vesterman, Eran Kotler, Sylvia Wilder, Lior Golomb, Ariel Pribluda and Ester Feldmesser, as well as Zohar Wolchinsky of the Technion – Israel Institute of Technology, Feng Zhang and Xiaochun Yu of the University of Michigan in the US, Mahmood Haj-Yahya and Ashraf Brik of Ben-Gurion University of the Negev, and Daniel Aberdam of the Technion and the University of Nice-Sophia Antipolis in France.
This study belongs to a relatively new direction in cancer research:
Rather than focusing on the genes involved, it highlights the role of epigenetics – that is, processes that do not modify the gene code, itself, but affect the way its information is interpreted within the cell. Understanding the epigenetic roots of cancer will advance the search for effective therapies for this disease.
Prof. Eytan Domany’s research is supported by the Kahn Family Research Center for Systems Biology of the Human Cell, which he heads; the Mario Negri Institute for Pharmacological Research - Weizmann Institute of Science Exchange Program; the Leir Charitable Foundations; and Mordechai Segal, Israel. Prof. Domany is the incumbent of the Henry J. Leir Professorial Chair.
Dr. Jacob Hanna’s research is supported by the Leona M. and Harry B. Helmsley Charitable Trust; Pascal and Ilana Mantoux, France/Israel; the Sir Charles Clore Research Prize; Erica A. Drake and Robert Drake; and the European Research Council.
Prof. Moshe Oren’s research is supported by the Dr. Miriam and Sheldon G. Adelson Medical Research Foundation; the Robert Bosch Foundation; the estate of Harold Z. Novak; and the European Research Council. Prof. Oren is the incumbent of the Andre Lwoff Professorial Chair in Molecular Biology.
Locally Produced Proteins
Several years ago, Prof. Michael Fainzilber and his group in the Biological Chemistry Department made a surprising discovery: Proteins thought to exist only near the cell nucleus could also be found in the far-off regions of the body’s longest cells – peripheral nerve cells that extend processes called axons, reaching up to a meter in length in adult humans.
These proteins, known as importins, have a well-studied role in the vicinity of the nucleus: They shuttle various molecules through the protective nuclear membrane. Fainzilber and his group showed that when a nerve cell is injured somewhere along its length, importins in the long axons hook into a sort of “railcar” mechanism, which then transports the “Help!” message from the injury site all the way to the nucleus.
These findings raised an intriguing question: How did importins get to the axons in the first place? Initial evidence suggested that one critical importin, called importin beta1, is produced locally upon injury near the site where it is needed. The problem was that years of scientific thinking on the subject indicated that proteins do not get manufactured in the axons, as investigations had turned up few of the cellular protein factories known as ribosomes there.
Settling the issue was far from simple: Importins are so crucial that even the smallest embryo could not survive without them. But Rotem Ben-Tov Perry, a joint research student in Fainzilber’s group and that of department colleague Dr. Avraham Yaron, found a way to distinguish the importin beta1 in the cell body from that in the axon: The axonal protein was apparently made from a longer messenger RNA. To see if they could selectively affect just the axonal version of the protein, the groups, together with Prof. Jeff Twiss of Drexel University, Philadelphia, Pennsylvania, took advantage of high precision knock-out technology. Rather than knocking a whole gene out of the system, they managed to remove one little piece of the messenger RNA that carries the encoded instructions for manufacturing importins: just the longer bit that sends the RNA to the axon.
Now they observed plenty of importin beta1 in the cell body, but none in the axons. Mice with the knocked out segment of RNA took much longer to recover from peripheral nerve injury, and the genes that are normally active in response to nerve damage were activated to a lesser degree. All of this suggests that the importin beta1 that normally helps inform the extended nerve cell about injury is, indeed, produced locally in the axon.
Fainzilber: “The data shows conclusively that importin beta1 protein is produced in axons, and Rotem’s work has validated the importins’ crucial role in nerve repair.” The findings, which appeared recently in Neuron, may help point the way toward better treatments for nerve damage and aid in finding ways to speed up the repair.
Prof. Michael Fainzilber’s research is supported by the Sylvia Schaefer Alzheimer’s Research Fund; the Kahn Family Research Center for Systems Biology of the Human Cell; the Dr. Miriam and Sheldon G. Adelson Medical Research Foundation; the Legacy Heritage Fund Program of the Israel Science Foundation; the Nella and Leon Benoziyo Center for Neurological Diseases; the Yeda-Sela Center for Basic Research; the estate of Raymond Lapon; the Irwin Green Alzheimer’s Research Fund; and the estate of Florence Cuevas. Prof. Fainzilber is the incumbent of the Chaya Professorial Chair in Molecular Neuroscience.
Dr. Avraham Yaron’s research is supported by the Wolfson Family Charitable Trust; the Koshland Family; the Rowland and Sylvia Schaefer Family Foundation; the Nella and Leon Benoziyo Center for Neurosciences; the Irving Harris Foundation; the estate of Lola Asseof; the estate of Nathan Baltor; and the Joseph D. Shane Fund for Neurosciences.