Tapping Particles of Light; Rare Disease is a Lens on Cancer; Sperm “See” It Hot
Tapping Particles of Light
Weizmann Institute scientists single out individual photons
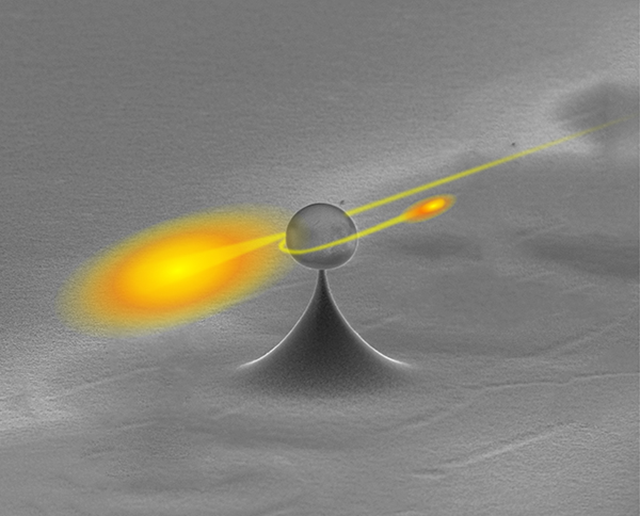
Capturing a single photon from a pulse of light: Devices based on the Weizmann Institute model may be the backbone of future quantum communications systems
At the Weizmann Institute of Science, researchers have managed to “pluck” a single photon – one particle of light – out of a pulse of light. The findings of this research, which appeared November 24 in Nature Photonics, bear both fundamental and practical significance: Light is the workhorse of today’s communication systems, and single photons are likely to be the backbone of future quantum communication systems. In addition, say the scientists, the apparatus they have devised will spur further research into the fundamental particle nature of light.
“Once we move over to quantum communication, information will have to be encoded in single photons,” says Dr. Barak Dayan, head of the Weizmann Institute Quantum Optics group. “Each photon will then represent a single ‘qubit’ – a quantum bit that can exist in more than one state at the same time – for example, an equal combination of both 1 and 0.”
Dr. Dayan and his research team, led by Dr. Serge Rosenblum and Orel Bechler, set out to demonstrate a scheme for pulling just one photon out of a stream, on demand. Their mechanism relies on a physical effect that they call single-photon Raman interaction, or SPRINT, which is based on a single atom, or atom-like system. “The advantage of SPRINT,” says Dr. Dayan, “is that it is completely passive – it does not require any control fields, just the interaction between the atom and the optical pulse.” In previous research, he and his team had employed SPRINT as a switch for single photons that sent them down different pathways, effectively turning the apparatus into a photonic router. In this work, the atom becomes a tap rather than a switch, snatching one photon from the flow and then turning itself off. “It is not trivial,” says Dr. Dayan, “to have a mechanism that continues to function even in high fluxes of photons and to remove just one photon.”
The experimental setup of Weizmann’s quantum optics group relies on state-of-the-art technologies: laser cooling and trapping of atoms (in this case, rubidium), the fabrication of chip-based, ultra-high-quality glass microspheres, and optical nanofibers.
“The ability to divert a single photon from a flow could be harnessed for various tasks,” says Dr. Dayan, “from creating nonclassical states of light that are useful for basic scientific research, through eavesdropping on imperfect quantum-cryptography systems that rely on single photons, to increasing the security of your own quantum-communication systems.”
The existence of photons was first suggested by Einstein in 1905, yet many of their properties are just now coming to light. Dr. Dayan believes the group’s new method will expand our capabilities to study and control photons as individual particles.
Dr. Barak Dayan’s research is supported by the Benoziyo Endowment Fund for the Advancement of Science; the Crown Photonics Center; the Rothschild Caesarea Foundation; and the Deloro Institute for Advanced Research in Space and Optics. Dr. Dayan is the incumbent of the Joseph and Celia Reskin Career Development Chair.
Rare Disease is a Lens on Cancer
In children, lack of a protein causes a dangerous disease. Why do cancer cells suppress the same protein?
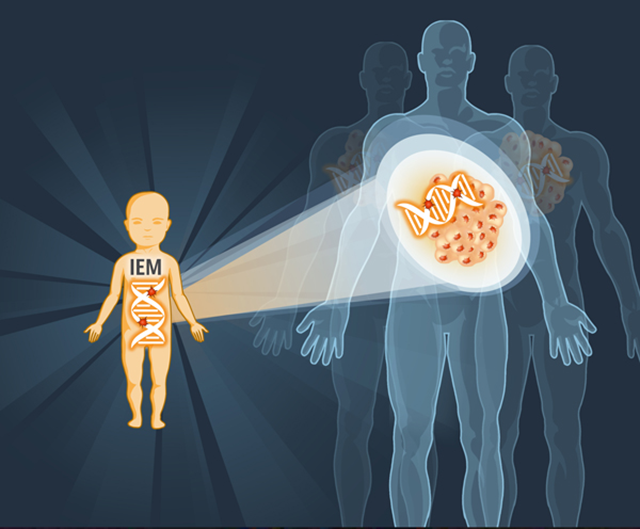
Learning what goes wrong with a specific gene that is involved in a rare childhood disease can also shed light on how that gene malfunctions in cancers
What can a rare genetic childhood disease teach us about cancer? Dr. Ayelet Erez of the Weizmann Institute of Science’s Department of Biological Regulation says: “A single-mutation disease can act as a ‘lens.’ If we find exactly what malfunctions in the sick child, we can zoom in and understand the role of the same gene among the many genetic changes that accompany cancer.” In findings that recently appeared in Nature, Dr. Erez and a team of researchers used this approach to reveal how a metabolic cycle that is “broken” in two childhood diseases gets hijacked to benefit cancer cells.
Dr. Erez, an MD/PhD, has treated children with a disease called citrullinemia. These children lack the activity of a protein known as ASS1; the absence of ASS1 leads to the toxic and, if untreated, fatal, buildup of ammonia in the body.
Many kinds of cancer prevent the expression of the ASS1 gene; silencing indicates a more aggressive cancer and a worse outcome. Yet efforts to develop treatments based on depleting the amino acid this gene produces have only had limited success.
Dr. Erez and her research group, led by graduate student Shiran Rabinovich, asked: What if the cancer cell, rather than silencing ASS1 because of what it makes, does so because of what it takes? Knowing that cancer cells often reroute the body’s normal metabolic pathways, they looked “upstream” – at the earlier connections of this protein in its metabolic cycle.
The team located an amino acid called aspartate in the metabolic pathway of ASS1; this amino acid is also required for the production of DNA and RNA. Now the connection was beginning to make sense: Cancer cells need to produce large amounts of DNA and RNA to keep dividing, so silencing ASS1 could be a way of freeing up the aspartate needed to meet the high demand.
An even rarer childhood disease – citrullinemia type II – gave the researchers an additional “therapeutic lens” on cancer. Here, rather than too much aspartate, there is too little, owing to the loss of another protein called citrin. Children with this disease tend to be smaller than average. “If the loss of citrin, and thus aspartate, could result in smaller children, we thought it might also help us achieve smaller tumors,” says Dr. Erez.
The team developed a method for blocking citrin and found that the growth of certain cancers was indeed inhibited by the treatment.
“There are hundreds of rare, hereditary disorders caused by mutations in single genes, and more than a few show up in such common diseases as cancer,” says Dr. Erez. “So investigating the rare can truly help shed light on the common.”
Also participating in this research were Drs. Lital Adler, Alona Sarver, Alon Silberman, and Shani Agron, all members of Dr. Erez’s lab; Dr. Keren Yizhak and Prof. Eytan Ruppin of Tel Aviv University; Drs. Qin Sun and Sandesh CS Nagamani of Baylor College of Medicine, Houston, Texas; Dr. Igor Ulitsky of the Weizmann Institute’s Department of Biological Regulation; and Dr. Shalev Itzkovitz of the Institute’s Department of Molecular Cell Biology.
Dr. Ayelet Erez’s research is supported by the Adelis Foundation; the Henry S. and Anne S. Reich Research Fund; Joseph Piko Baruch, Israel; the Dukler Fund for Cancer Research; the Paul Sparr Foundation; the estate of Fannie Sherr; and the Saul and Theresa Esman Foundation. Dr. Erez is the incumbent of the Leah Omenn Career Development Chair.
Sperm “See” It Hot
Proteins better known as visual sensors play a role in the heat-seeking movement of sperm
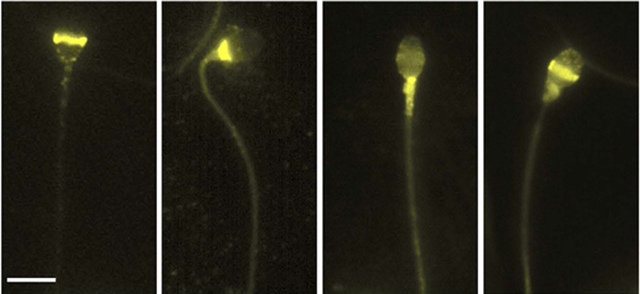
Locations of different opsins on the human sperm, viewed under a microscope, are revealed via labeling with a fluorescent antibody (bright yellow)
In their arduous journey to the egg, sperm “feel” the heat of the fallopian tube and “taste” the chemical signals of the ova. But a new Weizmann Institute of Science study published in Scientific Reports, in Nature, shows that sperm actually make quite a bit of use of sensors that have mainly been known to belong to the visual system.
If a sperm, about 46 microns (thousandths of a millimeter) long, were the size of a human being, it would swim several kilometers to reach its destination. Thus, sperm need to be guided by various cues.
In earlier studies, Prof. Michael Eisenbach and his team discovered two of these guidance mechanisms – heat sensing and chemical cues. The heat attraction works across the longer range: The site of the fertilization is warmer than the spot at the entrance to the fallopian tube where the sperm pause for maturation, and this temperature difference points them in the direction of the egg. When they get closer to the egg, they pick up its chemical signals. “As in all important processes in nature, the sperm rely on more than one mechanism in their navigation, so that if one breaks down, others can provide a backup,” Prof. Eisenbach says.
The heat sensitivity of sperm is extremely high. From a distance equivalent to the length of one sperm cell, they can sense differences in temperature as miniscule as 0.0006 of a degree Celsius – less than one thousandth of a degree. This sensitivity enables them to be guided by a very gradual increase in temperature on the way to the fertilization site.
In the new study, Prof. Eisenbach’s team – Dr. Serafín Pérez-Cerezales, Dr. Sergii Boryshpolets, Oshri Afanzar, Dr. Reinat Nevo, and Vladimir Kiss of the Department of Biological Chemistry and Dr. Alexander Brandis of Biological Services – set out to discover exactly how sperm sense the heat. The scientists examined a particular category of receptors that, based on their previous studies, were thought to be involved in conveying signals to the sperm. Within this category they zeroed in on a family of proteins called opsins.
Opsins are best known for their role in an entirely different sphere: the visual system. One major protein in this family, called rhodopsin, serves as a photoreceptor in the cells of the retina. But studies by other researchers working with fruit fly larvae had found this protein involved in responding to heat, hinting that this could also be the case in sperm.
The scientists found that several proteins in the opsin family of receptors were present on the surface of mouse and human sperm. Each opsin had its own distribution pattern on the sperm, and each apparently made a contribution, through its own set of signals, to heat sensing. When the researchers blocked the signals from these opsins, the sperm failed to swim from a cooler to a warmer chamber.
These rather surprising findings may explain the enigmatic presence of opsins in organs that are not exposed to light, including the lungs and the liver. They suggest that the mammalian opsins may be performing heat-sensing functions in these parts of the body.
Prof. Michael Eisenbach’s research is supported by the Benoziyo Endowment Fund for the Advancement of Science.